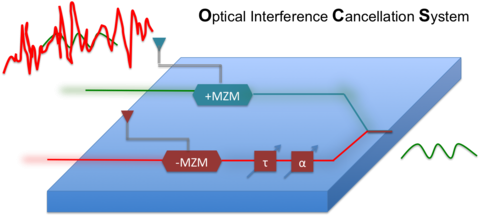
By converting radio frequency (RF) signals into the optical domain, it is possible to 'cancel' user-generated signals from the spectrum. For example, this system allows an aggressor to jam the communications of others while remaining unjammed. Waves from (a) the jamming signal (red) and (b) the surrounding environment (green+red) are converted into optical intensity fluctuations and subtracted from one another, resulting in the desired signal (green).
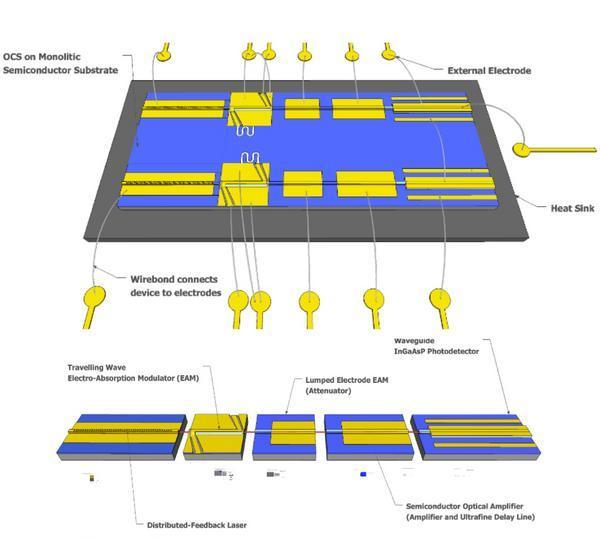
Illustration of an integrated photonic opto-cancellation system ready to be implemented in portable devices or cellphone radio towers. The footprint of an integrated system would be less than several square millimeters.
As the number of wireless devices grows exponentially in the coming decades, technologies will need to address the shrinking bandwidth availability of the radio frequency (RF) spectrum. One problem experienced by almost all RF devices is interference. A particular class of radio frequency (RF) interference, called co-site interference, occurs when a receiver cannot lock onto signals of interest because it is being overwhelmed by a much stronger simultaneous transmission from its own transmitting antenna. The problem is the reason why most of our communications today are half-duplex, leading to spectral and power inefficiencies. Interference cancellation is needed to realize the full potential of wireless communications. Traditional interference cancellation techniques are electronics-based, but their performance is consequently bandwidth-limited and precision-limited by the RF components. Electronic interference cancellation is also inhibited by the inability to cancel in-band interference. Our group has developed an optical technique for interference cancellation by using light to carry the RF information. The optical system, called the Opto-Cancellation System (OCS), is capable of cancelling both in-band interference as well as broadband signals over a huge bandwidth due to the low loss and high bandwidth of optics. To date, the OCS has demonstrated >70 dB cancellation of narrowband interference signals, as well as >30 dB cancellation over 40 MHz bandwidth in both the popular WiFi 900MHz and 2.4GHz frequency bands. This is 10x better than electronic methods while across a significantly broader bandwidth. The OCS is being developed for commercial applications with industry partner L3 Communications.
Journal Papers
J. Chang and P. R. Prucnal, "A novel analog photonic method for broadband multipath interference cancellation", IEEE Microwave and Wireless Component Letters, vol. 23, no. 7, pp. 377–379, Jul. 2013.
J. Bruno, M. Lu, Y. Deng, and P. R. Prucnal, “Broadband optical cosite interference cancellation,” Optical Engineering, vol. 52, no. 5, pp. 053001–053001, May. 2013.
M. Lu, M. P. Chang, Y. Deng, and P. R. Prucnal, “Performance comparison of optical interference cancellation system architectures,” Applied Optics, vol. 52, no. 11, pp. 2484–2493, Apr. 2013.
M. P. Chang, M. P. Fok, A. Hofmaier, and P. R. Prucnal, “Optical analog self-interference cancellation with electro-absorption modulators,” IEEE Microw. Wireless Compon Lett., vol. 23, no. 2, pp. 99–101, Feb. 2013.
Conference Papers
M. P Chang, D. Fisher, J. Wang, M. Lu, B. Chen, and P. R. Prucnal, “Microwave photonic self-interference cancellation system using a slow and fast light delay line,” in Proc. IEEE Photonics Conference (IPC), San Diego, CA, USA, Oct. 2014, paper TuC2.3.
M. Chang, A. Tait, J. Chang, and P. R. Prucnal, “An integrated optical interference cancellation system,” in Proc. Wireless Optical Communications Conference (WOCC), Newark, NJ, USA, May 2014. Paper O5.4.
M. P. Chang and P. R. Prucnal,“Amplitude-invariant fast light in a semiconductor optical amplifier for microwave photonics,” in Proc. Conference on Lasers and Electro-Optics (CLEO), San Jose, CA, USA, paper QM4E.3.
M. Lu, J. Bruno, Y. Deng, and P. R. Prucnal, and A. Hofmaier “Co-site interference mitigation using optical signal processing”, in Proc., vol. 8397, Baltimore, MD, USA, Apr. 2012, paper 839705.
Group Members:
- Paul Prucnal, Professor of Electrical Engineering
- Matt Chang, Graduate Student
Select Publications:
- Co-site interference mitigation using optical signal processing
- Suarez, J. So, S. Wysocki, G. and Prucnal, P., “Application of Counter-Phase Optical Interference Cancellation to Anti-Jamming in Wireless Sensor Networks”, IEEE Sensors Journal, submitted for publication, (2009).
- Suarez, J. and Prucnal, P., “System-Level Performance and Characterization of Counter-Phase Optical Interference Cancellation”, Journal of Lightwave Technology, Vol. 28, Issue 12, pp. 1821-1831 (2010).
- Suarez, J. Kravtsov, K. and Prucnal, P., “Incoherent Method of Optical Interference Cancellation for Radio-Frequency Communications”, IEEE Journal of Quantum Electronics, Vol. 45, Issue 4, pp. 402-408 (2009).
Broadband Adaptive Photonic Beamforming
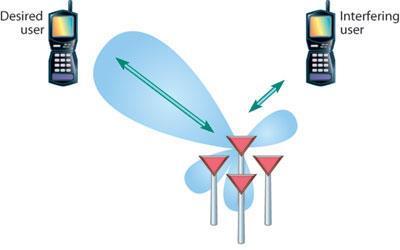
Encoding wireless signals on light waves allows antennas to selectively detect signals from a desired direction with performance levels that exceed what is possible with electronics.
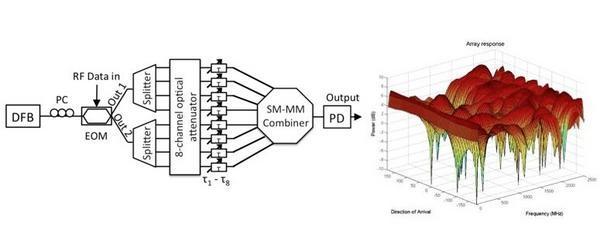
(Left) Response of precisely tuned beamformer as a function of the input direction and frequency. (Right) Schematic of a frequency response filter used for 'photonic beamforming.'
Traditional radio frequency (RF) communication systems involving direct broadcast and detection typically waste tranmission power, cause interference congestion, and lead to signal loss. One way of increasing the efficiency of the network is to send and receive signals directionally, preventing signals from overlapping with one another as they travel from source to receiver.
We have developed a technology called photonic beamforming that utilizes the unique physics of optics to create directionality in arrays of antennas. Systems including an array of antennas coupled to an adaptive processor can filter signals in both space and frequency, enabling the steering of a beam while rejecting interfering directional noise sources. Our broadband adaptive optical signal processing techniques can implement algorithms for real-time control of cognitive/adaptive antenna systems. For example, our novel control system will provide low-latency (nsec) reconfiguration of the antenna aperture to enable adapting to rapidly changing environments with varying clutter, tracking rapidly changing target characteristics (such as fast moving targets), and rapidly switching between different objectives (such as wide angle search and inspection where more detail about a target is extracted in a narrower field of view).
Book Chapters
B. J. Shastri*, J. Chang*, A. N. Tait, M. P. Chang, B. Wu, M. A. Nahmias, and P. R. Prucnal, “Ultrafast Optical Techniques for Communication Networks and Signal Processing,” in All-Optical Signal Processing for Data Communication and Storage Applications, Springer Berlin Heidelberg, 2014, ch. 11, 20 pages (in press). [*equal contribution].
Journal Papers
J. Chang, J. Meister, P. R. Prucnal, “Implementing a novel highly scalable adaptive photonic beamformer using “blind” guided accelerated random search", IEEE/OSAJournal of Lightwave Technology, vol. 32, no. 20, pp. 3623–3629, Oct. 2014.
J. Chang, M. P. Fok, R. M. Corey, J. Meister, and P. R. Prucnal, "Highly scalable adaptive photonic beamformer using a single mode to multimode optical combiner,” IEEE Microwave and Wireless Component Letters, vol. 23, no. 10, pp. 563–565, Oct. 2013.
J. Chang and P. R. Prucnal, "A novel analog photonic method for broadband multipath interference cancellation", IEEE Microwave and Wireless Component Letters, vol. 23, no. 7, pp. 377–379, Jul. 2013.
J. Chang, M. P. Fok, J. Meister, and P. R. Prucnal, "A single source microwave photonic filter using a novel single-mode fiber to multimode fiber coupling technique", Optics Express, vol. 21, no. 5, pp. 5585–5593, Jan. 2013.
J. Chang, Y. Deng, M. P. Fok, J. Meister, and P. R. Prucnal, “A photonic microwave FIR filter using a spectrally sliced supercontinuum source,” Applied Optics, vol. 51, no. 19, pp. 4265–4268, Jun. 2012.
Conference Papers
J. Chang, M. P. Fok, R. M. Corey, J. Meister, and P. R. Prucnal, "Highly scalable adaptive photonic beamformer using a single mode to multimode optical combiner,” IEEE Microwave and Wireless Component Letters, vol. 23, no. 10, pp. 563–565, Oct. 2013.
Group Members:
- Paul Prucnal, Professor of Electrical Engineering
- John Chang, Graduate Student
Microwave Photonic Linear Filters
Eric C. Blow – [email protected]
Microwave photonics is the cross section between microwave engineering and optoelectronics. The systems we explore are optical systems which process microwave signals for the radiofrequency (RF) domain. Initially, this technology was implemented in only the most extreme applications of RF electronics where traditional approaches were insufficient or at times impossible [1]. Current research demonstrates that the implementation of microwave photonics can be applied to standard RF systems such as filters, cancellers, and beamformers can enhance performance and enable novel applications [2]. The primary and most significant improvement is the vast bandwidth of photonics in both instantaneous bandwidth and tunability. The fractional bandwidth of a 10 GHz signal once upconverted to 193 THz becomes minute while compared to the fractional bandwidth of high-speed electronics.
While the bandwidth enhancement is tremendous, there are limitations of microwave photonics when analyzed with respect to traditional RF performance metrics. The primary RF limitations are loss and noise figure [3]. These undesirable metrics can be improved via improvements in device fabrication and designing better application specific architectures.
Integration of photonic circuits will vastly enhance the viability of these systems and extend the application reach of microwave photonics. While the endeavor to integrate photonic circuits started in the early 1990s, fabrication innovations have expedited the complexity capabilities of these circuits and ubiquitous deployment. Although photonic integration is in its infancy, the component complexity and density are following a Moore’s law curve like the development of integrated electronics [4]. These innovations are enabling better performance of microwave photonics and furthering the application space this technology can revolutionize.
This introduction to microwave photonics highlights the three research thrusts of the Lightwave Communication Research Lab’s microwave photonic subsection. To clearly reiterate, firstly, we focus on the exploration of microwave application regarding linear filters; primarily interference cancellation and beamforming. Secondly, we design and implement novel architectures to improve the RF performance metrics [5]. Lastly, we design and test microwave photonic integrated systems to dramatically reduce the size, weight, and power of our device [6].
Figures: (top left) Noise Figure Analysis of Integrated Microwave Photonic Canceller (IMPC); (bottom left) Example of Balanced IMPC; (right) Cancellation results of integrated IMPC
- Capmany, José, and Dalma Novak. "Microwave photonics combines two worlds." Nat. Phot. 1.6 (2007): 319.
- Seeds, Alwyn J., and Keith J. Williams. "Microwave photonics." JLT 24.12 (2006): 4628-4641.
- Cox, Charles H., et al. "Limits on the performance of RF-over-fiber links and their impact on device design." IEEE MTT 54.2 (2006): 906-920.
- Smit, Meint, J. J. G. M. Van der Tol, and Martin Hill. "Moore's law in photonics." LPR 6.1 (2012): 1-13.
- Blow, Eric C., et al. "Integrated Balanced Microwave Photonic Canceller" IPC 2018
- Chang, Matthew P., et al. "Integrated microwave photonic circuit for self-interference cancellation." IEEE MTT 65.11 (2017): 4493-4501.
Figures: (top left) Noise Figure Analysis of Integrated Microwave Photonic Canceller (IMPC); (bottom left) Example of Balanced IMPC; (right) Cancellation results of integrated IMPC
[1] Capmany, José, and Dalma Novak. "Microwave photonics combines two worlds." Nat. Phot. 1.6 (2007): 319.
[2] Seeds, Alwyn J., and Keith J. Williams. "Microwave photonics." JLT 24.12 (2006): 4628-4641.
[3] Cox, Charles H., et al. "Limits on the performance of RF-over-fiber links and their impact on device design." IEEE MTT 54.2 (2006): 906-920.
[4] Smit, Meint, J. J. G. M. Van der Tol, and Martin Hill. "Moore's law in photonics." LPR 6.1 (2012): 1-13.
[5] Blow, Eric C., et al. "Integrated Balanced Microwave Photonic Canceller" IPC 2018
[6] Chang, Matthew P., et al. "Integrated microwave photonic circuit for self-interference cancellation." IEEE MTT 65.11 (2017): 4493-4501.